Cliff Dwasieden
Please note: Once you watch the video, data will be transmitted to Youtube/Google. For more information, see Google Privacy.
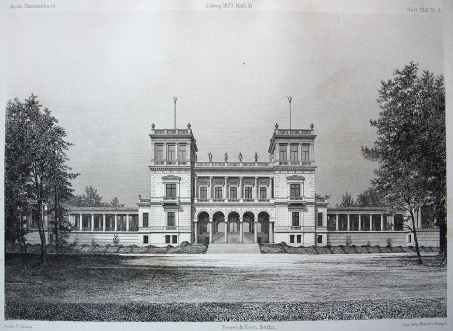
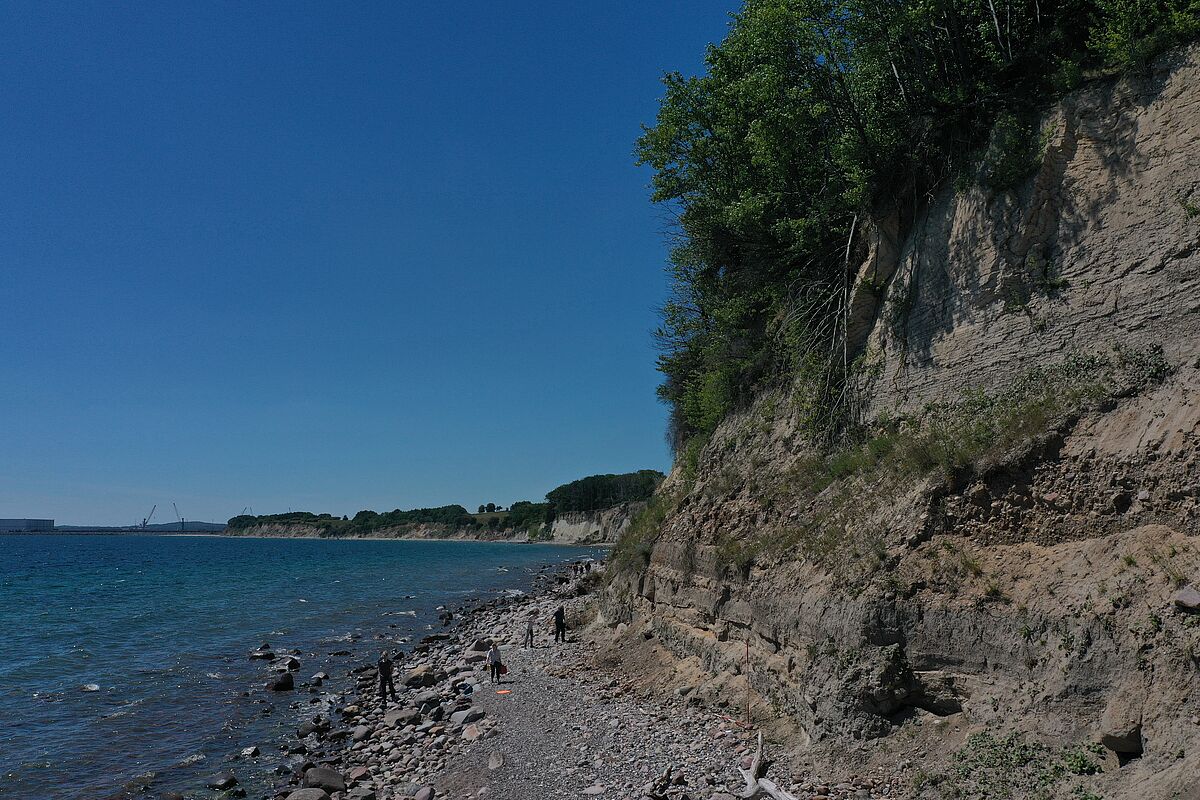
“We turn around, looking into the forest from the sea - there stands Dwasieden Castle in front of us! Yes, this is what Sleeping Beauty's palace must have looked like - surrounded by a dense forest, quiet and mysterious, rising to heaven like a poem."
(Autotype from: "Daheim dt. Familienblatt" 1895)
Dwasieden Castle was built between 1873 and 1877 and was considered the most valuable building on Rügen at the time (Fig. 1). The owner, Adolph von Hansemann, invested 4 million Reichsmark at the time and imported all the building materials by ship. Aisles specially built for this purpose were constructed in order to transport the heavy freight up the bluff. High-ranking personalities at that time, such as the German imperial couple or Gerhart Hauptmann, visited the neoclassical palace. From the 1930s, the former holiday residence was used as an officers' mess. In 1948 the building was finally blown up as a military legacy.
(Biederstaedt, 2021)
Dwasieden is known among geologists as an 800 m long section of cliffs (Fig. 2) on the Jasmund peninsula on Rügen between Mukran and Sassnitz. The advancing ice during the last glacial maximum (LGM) of the Vistula glaciation led to the formation of end moraines, which characterize the landscape of Jasmund today (Gehrmann & Harding, 2018). Ice Age deposits lie on the white chalk, which is significantly older at 68 million years. The glacial deposits consist of three layers of tills and intervening units of gravel, sand and clay. Unsorted deposits from a glacier are referred to as till. Together with the Cretaceous deposits, the older two till layers with the sediments in between were deformed, i.e. broken down into large shear slices and folds by the thrust of the ice. This is called a glacitectonic overprint. The youngest till layer was deposited on top of the entire complex only after deformation.
For those who are particularly interested
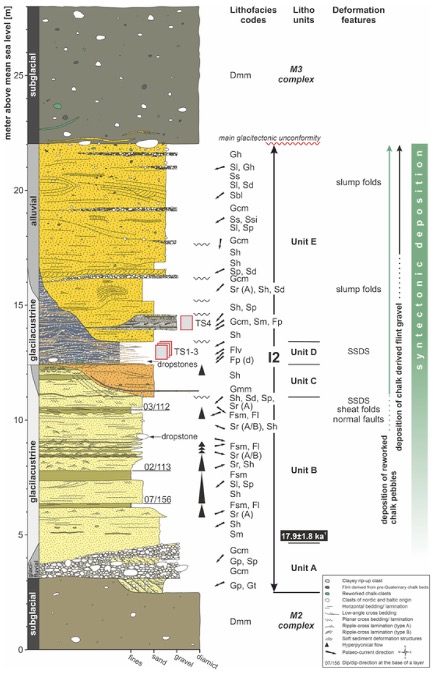
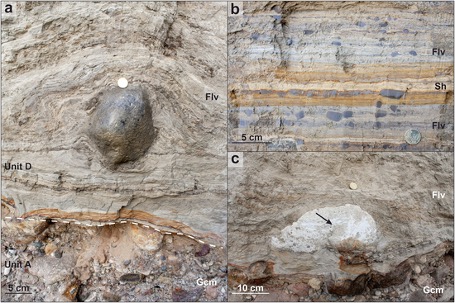
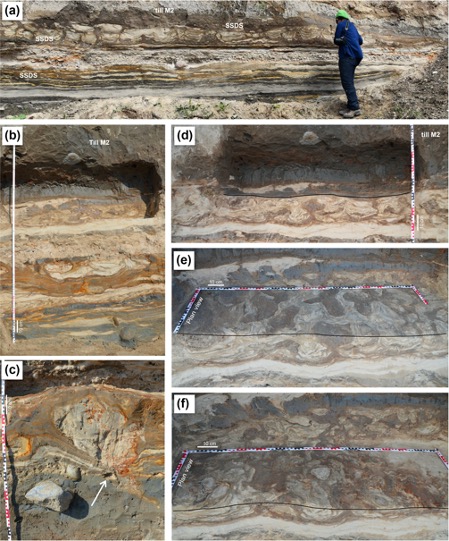
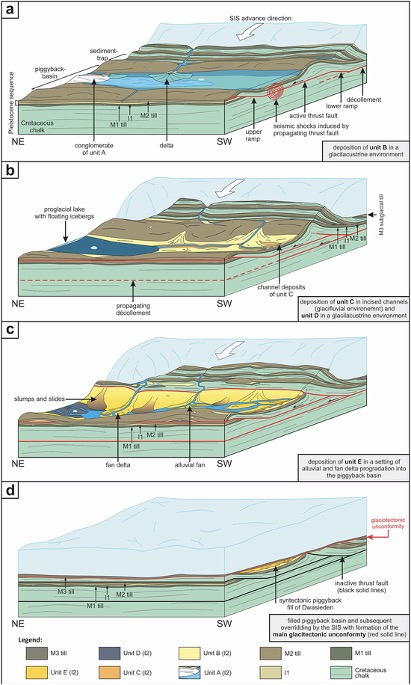
The ice age sediments deposited paraconformally, i.e. parallel to the chalk, consist of three glacial tills (M1, M2, M3) and intervening gravels, sands and clays (I1, I2) (Pisarska-Jamroży et al., 2018), which were formed in ice reservoirs (glacilimnic) or deposited by meltwater flows of the ice (glacifluviatile). The deposits of the I1, M2, I2 and M3 could be assigned to the Vistula glacial period by luminescence dating (method for absolute age determination) (Pisarska-Jamroży et al., 2018).
The oldest deposit is the chalk. The following 1.5 m thick till (M1) is characterized by a blue-grey color and its abundance of clasts. Through macroscale investigations, a NE dip of the longitudinal axes of pebble- to block-sized clasts could be identified (Brumme, 2019). The I1 sediments, characterized by horizontally layered fine sand, silt and clay, sometimes also contain layers of gravel with clasts up to the size of blocks. In contrast to the M1 till, the M2 till is low in clasts. According to Plonka et al. (2021) the following I2 sediments are divided into five lithological units (A-E) (Fig. 3), which reflect dynamic and rapidly changing depositional conditions.
Unit A is composed of glacifluvial sediments. This is followed by the 9.2 m thick glacial lacustrine sediments of Unit B. The fine to medium-grained, tabular layered sands are characterized by their horizontal and crossed ripple layers. These are overlain by massive layers of silt. This series is repeated within units C and D (Plonka et al., 2021). Unit C is unconformably overlain by trench-like structures on top of the sediments of Unit B. This 1.4 m thick unit is characterized by medium to coarse grain sands that are horizontal to obliquely stratified. Chalk clasts are abundant. Unit C is based on a matrix-supported gravel bed (Plonka et al. 2021). Unit D follows with a thickness of 5.8 m and is characterized by rhythmically stratified sediments with alternating layers from fine sand to clay. Layers with clay clasts are irregularly interposed (Fig. 4, Plonka et al. 2021). Unit E completes the I2 sequence with 8.6m thick alluvial sediments unconformably overlain by the M3 till.
Pisarska-Jamroży et al. (2018) describe deformed strata containing SSDS (soft-sediment deformation structures) within the sandy silts of the I1 unit (Fig. 5). SSDS are deformations in the fine- to medium-grained deposits that may have been caused by seismic liquefaction (Pisarska-Jamroży et al., 2019). For example, these structures can develop as a result of tremors caused by uplift processes (isostatic compensatory movements) after glaciation (neotectonics after glacier meltdown). This relation has already been described by different authors (e.g. Johnston, 1996; Muir-Wood, 2000; Kaufmann et al., 2005; Brandes et al., 2012; Hoffmann & Reicherter, 2012; Van Loon & Pisarska-Jamroży, 2014; Van Loon et al., 2016). However, it has not yet been sufficiently proven that an ice advance can also trigger earthquakes, as assumed in Dwasieden (Brandes et al., 2011; Pisarska-Jamroży et al., 2018, 2019).
During the genesis of the southern end moraine (glacitectonic complex) of Jasmund, so-called piggyback basins formed, which were filled with sediment at the same time (Müller & Obst, 2006; Kenzler et al., 2017; Pisarska-Jamroży et al., 2018; Kenzler & Hüneke, 2019). Piggyback basins can arise between two shear slices or between two folds (slope anticlines) (Pedersen, 2005; Pedersen & Boldreel, 2017; Plonka et al., 2021).
The gravel deposited in Unit A is indicative of the onset of the piggyback basin development (Fig. 6a). The transition from unit B to unit C, which is accompanied by stronger erosion, indicates complete drainage of the piggyback basin. The lowering of the erosion base (Fig. 6b) created the trench-like structures occurring in Unit B, which were refilled by the sediments of Unit C. A subsequently rising water level of the lake led to the deposition of unit D. So-called dropstones (Fig. 4a) indicate a lake with ice contact and icebergs floating in it. Subsequently, alluvial sediment fans developed due to higher deposition rates, reconstructable from unit E (Fig. 6c).
After the basin was filled, another ice advance followed and unconformably deposited the M3 till (Fig. 6d, Plonka et al., 2021). The chalk clasts contained in the M3 till indicate that the chalk must have been exposed (raised shear slices or folds) during the ice advance and thus exposed to erosion.
References
[Translate to English:]
Biederstaedt, F. (2021): HausGeist – Historische Rundwege in Sassnitz und Umgebung. Rundweg Nr. 5 – Dwasieden [zueletzt aufgerufen 13.10.2021].
Brandes, C., Polom, U., Winsemann, J. (2011): Reactivation of basement faults:
interplay of ice-sheet advance, glacial lake formation and sediment loading, Basin Res., 23, 53–64.
Brandes, C., Winsemann, J., Roskosch, J., Meinsen, J., Tanner, D. C., Frechen, M.,
Steffen, H., Wu, P. (2012): Activity along the Osning Thrust in central Europe during the Lateglacial: Ice-sheet and lithosphere interactions, Quaternary Sci. Rev., 38, 49–62.
Brumme, J. (2015): Three-dimensional microfabric analyses of Pleistocene tills from the cliff section Dwasieden on Rügen (Baltic Sea Coast): Micromorphological evidence for subglacial polyphase deformation, Doctoral thesis, University of Greifswald, 1–250.
Brumme, J., Hüneke, H., Phillips, E. (2019): Micromorphology and clast microfabrics of subglacial traction tills at the sea cliff Dwasieden: evidence of polyphase syn- and post-depositional deformation. Deuqua Spec. Pub. 2, 51-60.
Gehrmann, A., Harding, C. (2018): Geomorphological mapping and spatial analyses of an Upper Weichselian glacitectonic complex based on LiDAR data, Jasmund Peninsula (NE Rügen), Germany. Geosciences 8 (6), 208.
Hoffmann, G., Reicherter, K. (2012): Soft-sediment deformation of Late Pleistocene
sediments along the southwestern coast of the Baltic Sea (NE Germany), Int. J. Earth Sci., 101, 351–363.
Johnston, A. C. (1996): A Wave in the Earth, Science, 274, 735.
Kaufmann, G., Wu, P., Ivins, E. R. (2005): Lateral viscosity variations beneath
Antarctica and their implications on regional rebound motions and seismotectonics, J. Geodynam., 39, 165–181.
Kenzler, M., Hüneke, H. , 2019. Sea cliff at Glowe: stratigraphy and absolute age chronology of the Jasmund Pleistocene sedimentary record. DEUQUA Spec. Pub. 2, 43–50.
Kenzler, M., Tsukamoto, S., Meng, S., Frechen, M., Hüneke, H. , 2017. New age
constraints from the SW Baltic Sea area – implications for Scandinavian Ice Sheet dynamics and palaeoenvironmental conditions during MIS 3 and early MIS 2. Boreas 46 (1), 34–52.
Kenzler, M., Tsukamoto, S., Meng, S., Thiel, C. , Frechen, M., Hüneke, H. , 2015.
Luminescence dating of Weichselian interstadial sediments from the German Baltic Sea coast. Quat. Geochronol. 30, 215–256.
Ludwig, A. O. (1954/55): Eistektonik und echte Tektonik in Ost-Rügen (Jasmund),
Wissenschaftliche Zeitschrift Universität Greifswald, 4, 251–288.
Ludwig, A. O., Panzig, W.-A. (2010): Stopp 5: Das Pleistozän südlich Sassnitz – Fazies Und Lagerung glazilimnischer/- fluviatiler Sedimente am Kliff bei Dwasieden, in: Eiszeitlandschaften in Mecklenburg-Vorpommern, edited by: Lampe, R. and Lorenz, S., 68 69, Geozon, Greifswald. Murton, J. B.: Near-Surface Brecciation
Mörner, N. A. (1990): Glacioisostatic and long-term crustal movements in Fennoscandia with respect to lithospheric and atmospheric processes and properties, Tectonophysics, 176, 13–24.
Muir-Wood, R. (2000): Deglaciation seismotectonics: a principal influence on intraplate seismogenesis at high latitudes, Quaternary Sci. Rev., 19, 1399–1411.
Müller, U., Obst, K., 2006. Lithostratigraphie und Lagerungsverhältnisse der pleistozänen Schichten im Gebiet von Lohme (Jasmund/Rügen). Z. Geol. Wiss. 34, 39–54 (in Germ an).
Ori, G.G., Friend, P.F., 1984. Sedimentary basins formed and carried piggyback on active thrust sheets. Geology 12 (8), 475–478.
Panzig, W.-A., 1995. Zum pleistozän von Rügen. Terra Nostra (Bonn) 6, 177–200 (in German).
Pedersen, S.A.S., 2005. Structural analysis of the Rubjerg Knude glaciotectonic complex, Vendsyssel, northern Denmark. Geol. Surv. Den. Greenl. Bull. 8.
Pedersen, S.A.S., Boldreel, L.O., 2017. Glaciotectonic deform ations in the Jammerbugt and glaciodynamic development in the eastern North Sea. J. Quat. Sci. 32 (2), 183–195.
Phillips, E., Spagnolo, M., Pilmer, A. C. J., Rea B. R., Piotrowski, J. A., Ely, J. C.,
Carr, S. (2018): Progressive ductile shearing during till accretion within the deforming bed of a palaeo-ice stream, Quaternary Sci. Rev., 193, 1–23.
Pisarska-Jamrozy, M., Belzyt, S., Börner, A., Hoffmann, G., Hüneke, H., Kenzler, M., Obst, K., Rother, H., van Loon, A. J. (2018): Evidence from seismites for glacio-isostatically induced crustal faulting in front of an advancing land-ice mass (Rügen Island, SW Baltic Sea), Tectonophysics, 745, 338–348.
van der Meer, J. J. M. (1993): Microscopic Evidence of Subglacial Deformation,
Quarternary Sci. Rev., 12, 553–587.
van Loon, A. J. (2009): Soft-sediment deformation structures in siliciclastic sediments: an overview, Geologos, 15, 3–55.
van Loon, A. J., Pisarska-Jamrozy, M. (2014): Sedimentological evidence of Pleistocene earthquakes in NW Poland induced by glacio-isostatic rebound, Sediment. Geol., 300, 1–10.
van Loon, A. J., Pisarska-Jamrozy, M., Nartišs, M., Krievans, M., Soms, J. (2016):
Seismites resulting from high-frequency, highmagnitude earthquakes in Latvia caused by Late Glacial glacioisostatic uplift, Journal of Palaeogeography, 5, 363–380.
Waller, R., Phillips, E., Murton, J., Lee, J., Whiteman, C. (2011): Sand intraclasts as evidence of subglacial deformation of Middle Pleistocene permafrost, North Norfolk, UK, Quaternary Sci. Rev., 30, 3481–3500.